Revealing the interlayer van der Waals coupling of epitaxially-grown few layers MoS2 by terahertz coherent phonon spectroscopy
Professor Chi-Kuang Sun
Graduate Institute of Photonics and Optoelectronics, National Taiwan University
台湾大学光电所 孙启光教授
Semiconducting 2D materials such as layered-stacking MoS2 were widely considered as a versatile platform for electronics design and for novel physics research. Due to their excellent electronic and optical properties, the direct and large area synthesis method for few-layer MoS2 is in demand. However, numerous studies have reported that the van der Waals (vdWs) interfaces are commonly defective, and thus it is crucial to have a non-destructive detection technique to monitor and ensure the high interfacial quality. In this work, we employed picosecond ultrasonics technique to observe the actual interlayer vdWs bonding strength. Since the observed physical quantity is determined by the electronic behavior of the 2D structure, it serve as a good indicator for the performance of these devices.
We first applied THz coherent phonon spectroscopy to optically probe the vibrational modes of the epitaxially-grown bi-layer and tri-layer MoS2. The interlayer out-of-plane vibrations beyond 1 THz were stimulated and temporally retrieved by femtosecond laser pulses (see Fig. 1(a)), revealing both Raman-active and Raman-inactive modes in one measurement (see Fig. 1 (b)). With the complete breathing modes revealed, here we extend the linear chain model by considering the elastic contact with the substrate and the vdWs coupling of next-nearest-layer to analyze the effective spring constants. We further considered the intralayer stiffness as a correction term to acquire the actual interlayer vdWs coupling. Our THz phonon spectroscopy results provide the interlayer spring constants for bi-layer and tri-layer, and a softening effect was observed when layer number decreases. The extended model further suggests that a non-negligible substrate mechanical coupling and the next-nearest-neighbor vdWs coupling have to be considered, and both of them are responsible for the correction of the large deviation when comparing with the lowest Raman active mode (see Fig. 2).
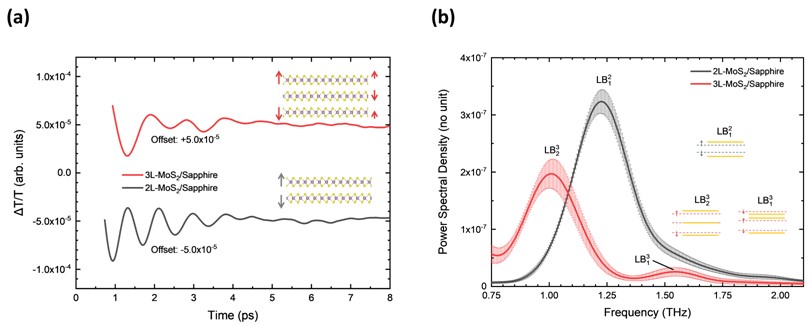
|
Fig. 1. (a) The measured interlayer-resonance-induced optical transmission change of bi-layer and tri-layer MoS2, showing a decaying negative cosinusoidal displacement of the layers; (b) Coherent phonon spectra of the bi-layer (black line) and tri-layer (red line) MoS2. Contents of this figure has been published on the Photoacoustics (2022) (see ref [1]).
|
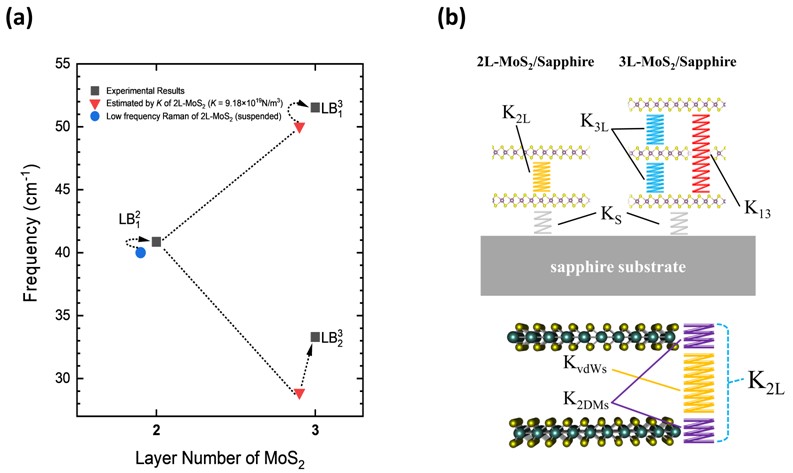
|
Fig. 2. (a) The fan diagram of LB modes of bi-layer and tri-layer MoS2 from the experiments, cited reference for low-frequency Raman result, and the simple LCM model prediction; (b) Schematically illustrating the complete models with interlayer vdWs coupling, next nearest neighbour effect, substrate’s mechanical coupling (top), and intralayer stiffness (bottom) considered. Contents of this figure has been published on the Photoacoustics (2022) (see ref [1]).
|
Reference:
[1] P.-J. Wang, P.-C. Tsai, Z.-S. Yang, S.-Y. Lin, C.-K. Sun, "Revealing the interlayer van der Waals coupling of bi-layer and tri-layer MoS2 using terahertz coherent phonon spectroscopy," Photoacoustics 28 (2022)
Design and Analysis of Integral Imaging based 3D Light-Field Display
Professor Hoang-Yan Lin
Graduate Institute of Photonics and Optoelectronics, National Taiwan University
台湾大学光电所 林晃岩教授
The integral-imaging based 3D light field display can restore its light-field information, reconstruct different images in different depth planes, and realize the floating projection effect. In the metaverse, AR/VR wearable devices, HUD for smart cockpits, medical care, entertainment, art performance and other scenarios, naked-eye 3D display is one of the most crucial requirements for the coming future.
Using the Computer Generated Elemental Image (CGEI) algorithm, we realize the generation of elemental image from the 2D image and its depth map, and use the ray-tracing method to simulate and analyze the reconstructed planes of different depths. Finally, naked eye 3D display and VAC free can be achieved.
Fig. 1 shows the design principle of the CGEI algorithm. Using the reversibility of light, the floating image can be restored during the display process. We input an image of ABC, and set the depth of "A" to 200 mm, the depth of "B" to 105.37 mm, and the depth of “C” to 48 mm. Using the CGEI algorithm, the elemental image is calculated as follows in Fig. 2. Considering the real display environment, we use the Monte Carlo ray tracing method of the software LightToolsTM for simulation and analysis. We set the receiver depth to 20 mm, 48 mm (“C”), 80 mm, 105.37 mm (“B”), 150 mm, 200 mm (“A”), and the results are shown in Fig 3.
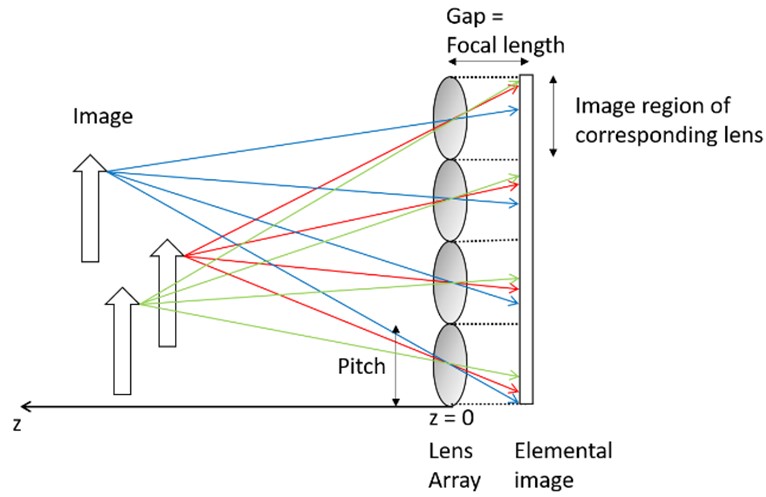
|
Fig. 1. Design principle of CGEI algorithm.
|
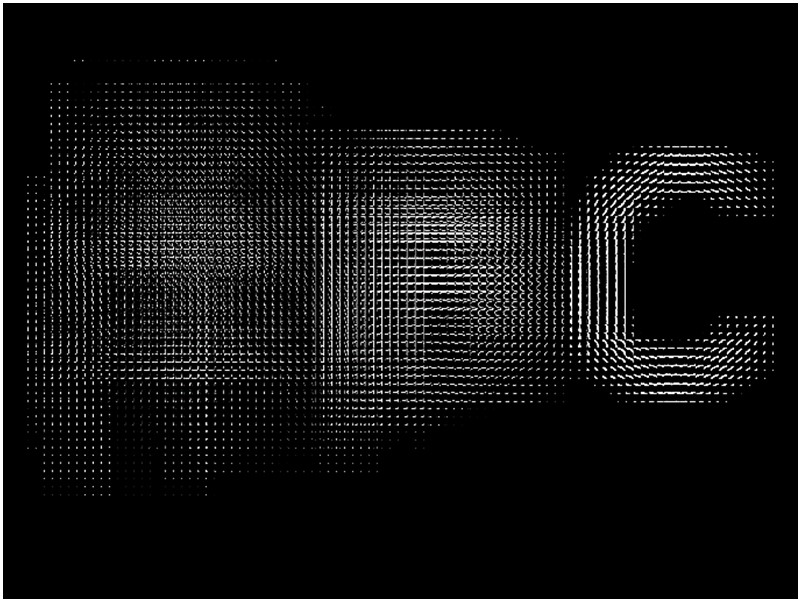
|
Fig. 2. Calculated elemental images.
|
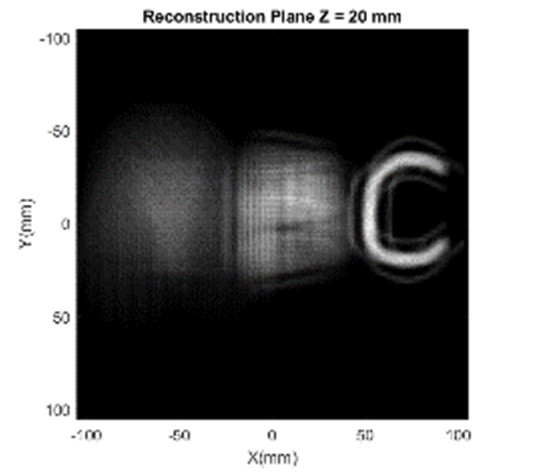
|
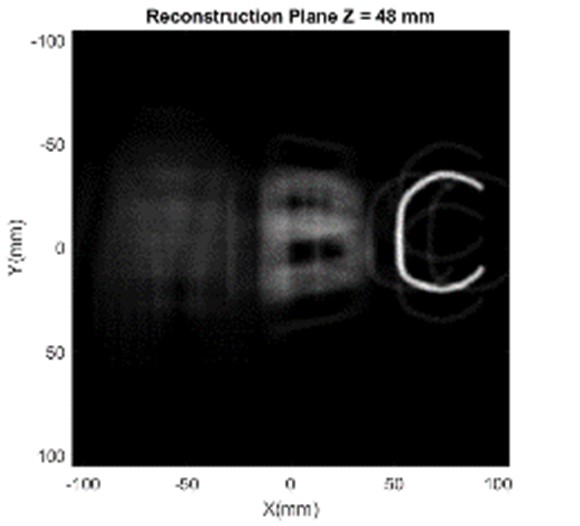
|
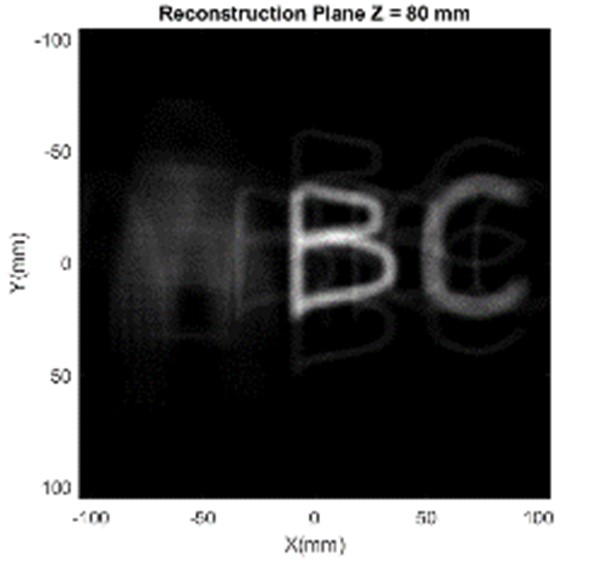
|
(a) z = 20mm
|
(b) z = 48mm
|
(c) z = 80mm
|
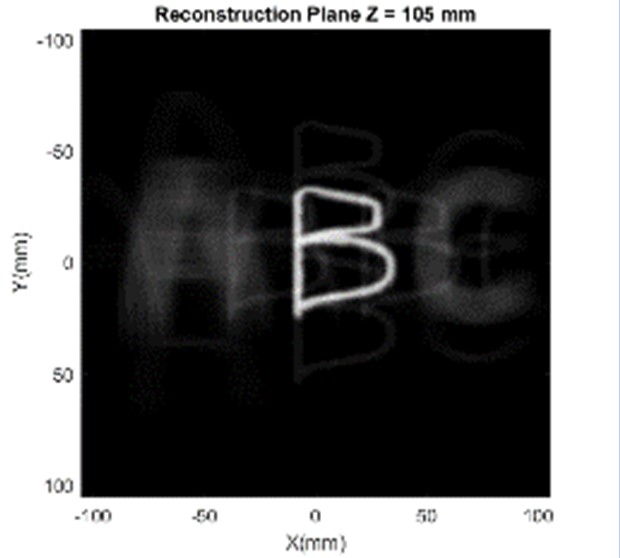
|
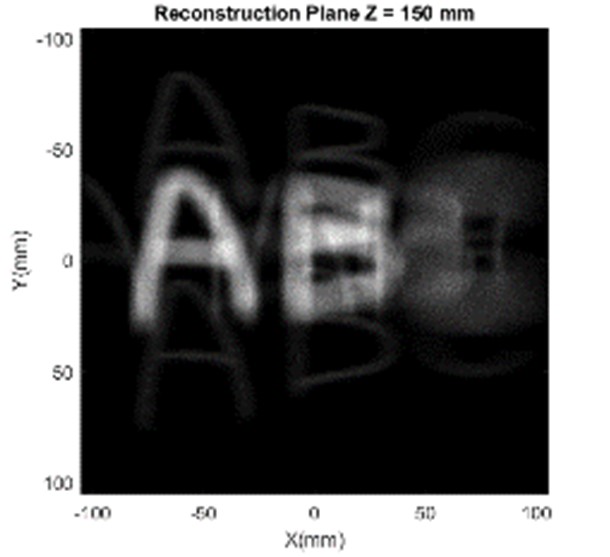
|
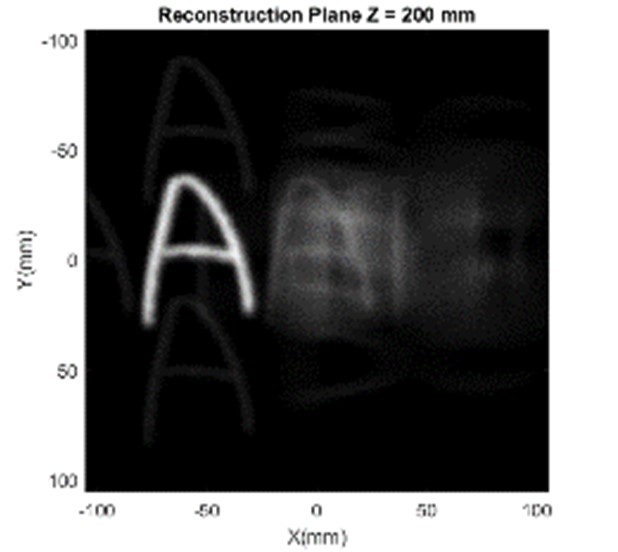
|
(d) z = 105.37mm
|
(e) z = 150mm
|
(f) z = 200mm
|
Fig. 3. Images at reconstruction planes of different depths.
|
Reference: Kai-Siang Hsu, Chia-Yuan Chang, and Hoang-Yan Lin, "Design and Analysis of Integral Imaging based 3D Light-Field Display," IDW 2022.
|