 |
|
 |
|
发行人:黄建璋所长 编辑委员:曾雪峰教授 主编:林筱文 发行日期:2021.04.30 |
|
|
 |
本所5、6月份演讲公告:
日期 |
讲者 |
讲题 |
地点 |
时间 |
5/7 |
孙庆成讲座教授
国立中央大学光电系
2020年中华民国光电学会理事长 |
光学新视界 |
博理馆 101演讲厅 |
14:20~16:00 |
5/14 |
蔡富吉执行长
Chief executive officer, 澔心科技股份有限公司 |
待订 |
博理馆 101演讲厅 |
14:20~16:00 |
5/21 |
陈阶晓执行长
钛隼生物科技股份有限公司 |
Start-up
Company and Our Robotic Application |
博理馆 101演讲厅 |
14:20~16:00 |
5/28 |
张鼎张讲座教授
国立中山大学物理系 |
低温缺陷钝化技术在半导体组件应用 |
博理馆 101演讲厅
|
14:20~16:00 |
6/11 |
瑞鼎科技股份有限公司 |
待订 |
博理馆 101演讲厅 |
14:20~16:00 |
|
|
 |
|
 |
|
|
|
 |
3月份「光电所专题演讲」(整理:姚力琪) |
时间: |
2021年3月12日(星期五)下午2时20分 |
讲者: |
刘致为特聘教授(国立台湾大学光电所) |
讲题: |
3Dx3Dx3D: Moore's Law continuing |
 |
本场演讲者刘致为特聘教授 |
|
时间: |
2021年3月19日(星期五)下午2时20分 |
讲者: |
吴志凌副处长(镎创科技先进技术开发处) |
讲题: |
PlayNitride MicroLED Display - PixeLED® Display Technology |
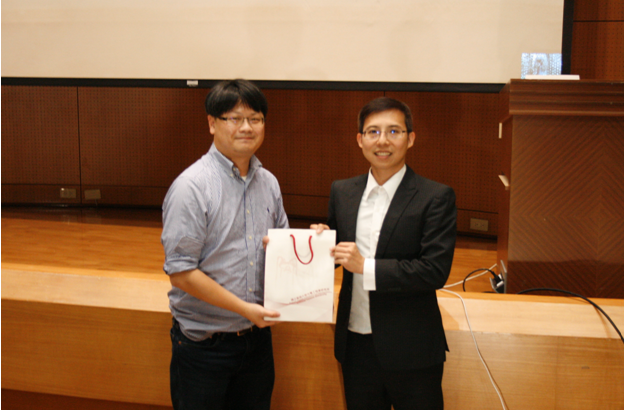 |
吴志凌副处长(右)与本所李翔杰教授(左)合影 |
|
时间: |
2021年3月26日(星期五)下午2时20分 |
讲者: |
李正匡博士(NVIDIA AI Technology Center) |
讲题: |
Accelerating AI and HPC – NVIDIA computing platform |
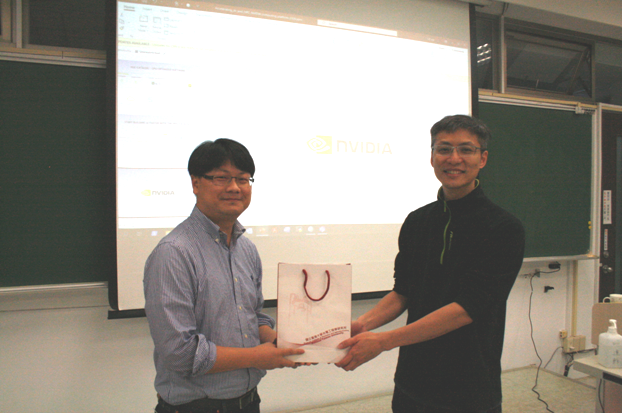 |
李正匡博士(右)与本所李翔杰教授(左)合影 |
|
4月份「光电所专题演讲」(整理:姚力琪) |
时间: |
2021年4月16日(星期五)下午2时20分 |
讲者: |
徐茂杰博士(Luxnet 华星光通技术长) |
讲题: |
半导体激光在光通应用的发展趋势 |
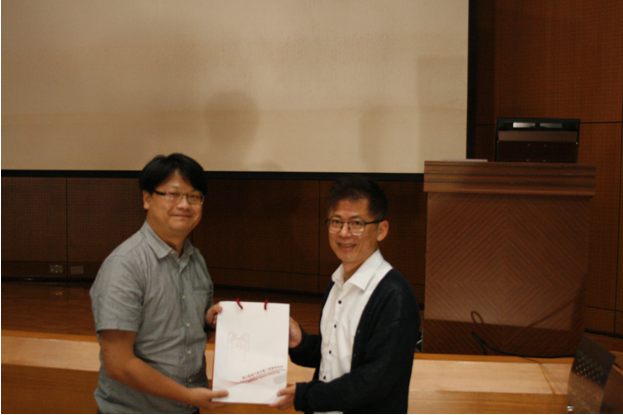 |
徐茂杰博士(右)与本所李翔杰教授(左)合影 |
|
时间: |
2021年4月23日(星期五)下午2时20分 |
讲者: |
林贞宏博士(佳世达科技股份有限公司) |
讲题: |
科技/艺术&设计/优化,从光学开始的奇幻旅程 |
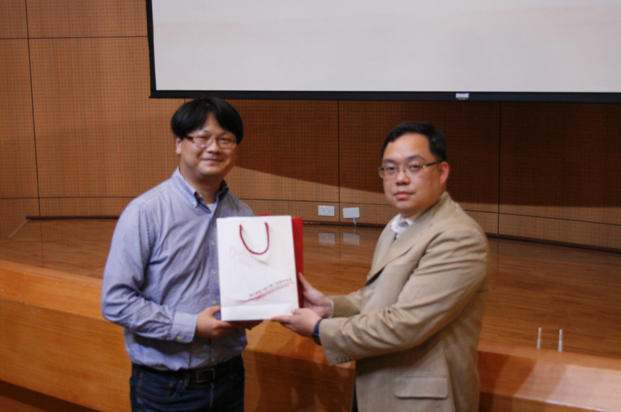 |
林贞宏博士(右)与本所李翔杰教授(左)合影 |
|
|
|
|
 |
|
 |
|
|
|
 |
Characterizations of Protein-Ligand Reaction Kinetics by Transistor-Microfluidic Integrated Sensors
Professor Jian-Jang Huang’s Lab
Graduate Institute of Photonics and
Optoelectronics, National Taiwan University
台湾大学光电所 黄建璋教授
Understanding the binding affinities and kinetics of protein-ligand interactions using a label-free method is crucial for identifying therapeutic candidates in clinical diagnostics and drug development. In this work, the IGZO-TFT (thin-film transistor) biosensor integrated with a tailored microfluidic chip was developed to explore binding kinetics of protein-ligand biochemical interactions in the real-time manner. The IGZO-TFT sensor extracts the binding characteristics through sensing biomolecules by their electrical charges. Using lysozyme and tri-N-acetyl-D-glucosamine (NAG3) as an example, we established a procedure to obtain the parameters, such as the dissociation constant, Kd, and association rate constant, ka, that are critical to biochemical reactions. The correlation between the lysozyme concentration and TFT drain current signal was first constructed. Next, solutions of lysozyme and NAG3 of different mixing ratios were prepared. They were pre-mixed for various periods of reaction time before applying to the TFT sensor to extract signals of lysozyme molecules and the concentration remaining. With the knowledge of drain current changes at different reaction times, ka and Kd can be obtained. The values from our experiment are comparable to other methods, which suggests the proposed approach can be employed to explore protein-ligand interaction kinetics in the massively parallel manner if the TFT array is considered.
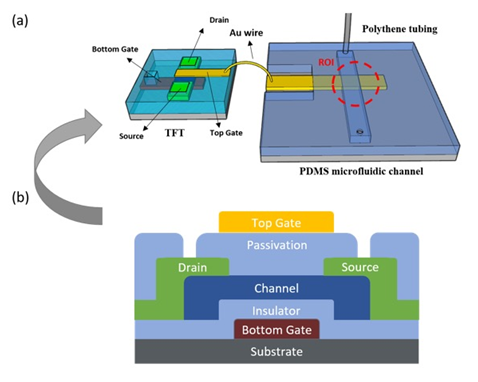
|
Fig. 1. (a) Schematic diagram of the IGZO-TFT biosensor integrated with a microfluidic channel. (b) Cross sectional view of the staggered and double-gated TFT. |
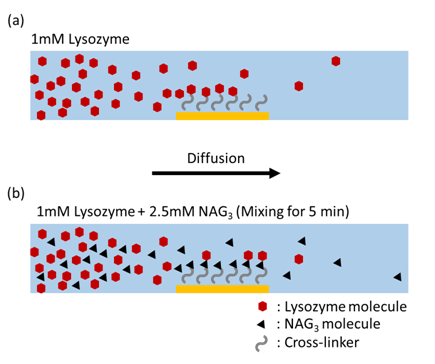
|
Fig. 2 Illustration of molecule capture process of (a) Lysozyme solution (b) lysozyme + NAG3 solution. Note that in addition to the screening effect, with the presence of NAG3, the drain current will be increased instead of decreased as opposed to the case of purelysozyme solution. |
Flexible Complementary Oxide Thin-Film Transistor-Based Inverter
with High Gain
Professor I-Chun Cheng
Graduate Institute of Photonics and
Optoelectronics, National Taiwan University
台湾大学光电所
陈奕君教授
Wearable bio-sensing devices are considered promising for ubiquitous heath monitoring. To accurately read out small bio-signals, the development of high-performance flexible front-end circuits is crucial. Oxide semiconductors are considered one of the most promising active channel materials for on-polymeric-foil electronics. So far, most flexible complementary TFT inverters have been based on either pseudo-CMOS or organic-inorganic hybrid-CMOS configurations. In this study, a high-gain flexible CMOS inverter with a beta ratio (width-to-length ratio between the pull-up and pull-down transistors) of 1 was demonstrated by monolithically integrating a top-gated n-type IGZO TFT and a bottom-gated p-type SnO TFT on a 5.5-μm-thick polyimide substrate, as shown in Figure 1. The IGZO TFT exhibits a field-effect mobility of 8.0 cm2V-1s-1, threshold voltage of 5.2 V, subthreshold swing of 0.15 V/decade, and high on/off current ratio of >107. The corresponding values for the SnO TFT are 1.6 cm2V-1s-1, 2.8 V, 2.3 V/decade and >103, respectively. No apparent current crowding is observed in the output characteristics, suggesting that ohmic contacts exist between the source/drain electrodes and the channel layers. The flexible inverter exhibited a high static voltage gain of 370 V/V and balanced noise margins (noise margin high of 4.8 V, noise margin low of 4.7 V) for a supply voltage of 10 V, as shown in Figure 2(a). In addition, as shown in Figure 2(b), the voltage transfer characteristics remained virtually unchanged when the device was subjected to an outward or an inward bending radius of 2.5 mm, indicating the complementary oxide-TFT-based inverter is practical for flexible electronics applications. [IEEE Trans. Electron Devices, vol. 68, No. 3, p. 1070, Mar 2021]
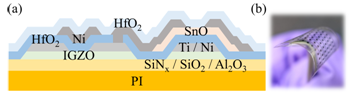
|
Fig. 1 (a) Schematic cross section of a flexible oxide-TFT-based CMOS inverter. (b) Photograph of flexible CMOS inverters fabricated on an ultrathin polyimide substrate. |
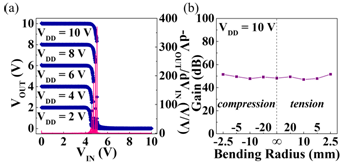
|
Fig. 2 (a) Voltage transfer characteristics of a flexible CMOS inverter with a beta ratio of 1 at different VDD. (b) Static voltage gain of the flexible CMOS inverter under different bending conditions. |
|
 |
|
|
|
|
 |
|
|
|
 |
论文题目:近红外宽带之单模晶体光纤光源研究
姓名:杨腾毅 指导教授:黄升龙教授
摘要 |
本论文两大主轴,一主轴为研发Cr4+:Y2SiO5做为近红外宽带晶纤光纤激光材料,另外一个主轴为使用固态单晶生长纤衣法做成单模态晶体光纤激光。过程中有观察到Cr4+:Y2SiO5的旋节分解。在每分钟30公厘生长速度,旋节分解面积比例会降低到0.4%。使用硼硅酸盐玻璃作为纤衣,半高宽带谱可以达到246 奈米,中心波长为1257奈米,相较于Cr4+:YAG中心波长1380奈米,这样放光波段因避开水吸收,且降低散射,而提高穿透深度,所以非常适合作为光学同调断层扫描术光源。估算纵向分辨率在自由空间中为 2.8微米,而其在生物组织内约为2微米。
本论文另外一主轴为Ti:Al2O3单模态晶体光纤生长和特性检测,使用浸涂法和摄氏1750度高温烧结来生长纤衣。观察到在单晶纤心周围有固态单晶生长产生,而纤衣在沿着(01 ̅1)生长为2.7倍于(001)生长方向。使用背向散射电子绕射技术和电子显微镜可以确定其为单晶结构。在光学量测中,使用穿透光的远场分布跟单模光场分布吻合,如图一所示,可以证实其为单模态晶体光纤。使用的纤心为30微米直径,折射差为1.0 x 10-4。在荧光频谱在端面量测里,可以观察到三价钛离子良好的局限于纤心于单晶纤衣生长过程,如图二所示。
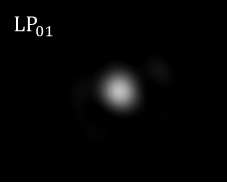 |
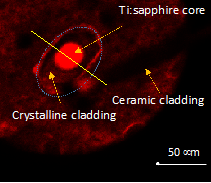 |
图一、穿透光远场图 |
图二、端面荧光影像 |
|
|
 |
|
 |
|
|
|
 |
— 资料提供:影像显示科技知识平台 (DTKP, Display Technology
Knowledge Platform) —
—
整理:林晃岩教授、卓真禾 —
原子尺度的波形采样
原子尺度与超快速时间标度的物质操纵会发生在重要过程中,例如电子转移,分子运动和化学反应等。这些时空动力学的可视化是科学界的长期目标。近年来,场驱动奈米影像技术是奈米光子学的一个新兴领域,它为研究具有极高时空分辨率的光-物质相互作用的非微扰机制开辟了新途径。由于这种光-物质交互作用不仅对电场的幅度而且对相位都敏感,因此精确控制超短激光脉冲的载波包相位(carrier-envelope phase, CEP)极为重要。
激光技术的最新进展使高精度超短激光脉冲的CEP控制达成可能,并且使用这种脉冲,许多研究成功地证明了在各种定制的奈米结构中电子的超快速相干控制1,2。在这种情况下,透过将持续时间只有几个光学周期的激光脉冲耦合到奈米结构,电场就被限制在超过光学绕射极限的奈米尺度上,而增强的场可以在次波长尺度和超快时间标度内探索光与物质的相互作用。
结果,光驱动的扫描穿隧显微镜(scanning tunnelling microscopy, STM)使得探索次分子分辨率的光-物质交互作用成为可能3–6。特别是,太赫兹场驱动的STM(THz-STM)4已用于追踪奈米级的载子动力学4,5和分子运动6。透过调整单周期太赫兹脉冲的CEP,太赫兹近场波形可以在单个隧道接点处同调地诱导电子穿隧,从探针探针尖端到样品,或反之亦然7。在这些实验中,使用电光采样来评估接点处的THz近场波形。但是,由于太赫兹近场的形状与太赫兹远场的形状完全不同8,因此非常需要在实际的直接测量中取得太赫兹近场波形。为此,已经提出了几种方法,例如使用太赫兹相位调整器9和光子辅助隧穿10 。尽管这些技术对于在扫描穿隧显微镜的尖端与样本配置中以表征CEP和近场波形很有用,但是在原子尺度上仍未实现无参数方法来定量评估电磁近场波形,这对于超快奈米科学和奈米电子学的进一步发展必不可少。
现在,多米尼克•佩勒(Dominik Peller)和同事在《自然光子学》(Nature Photonics)上发表了一篇文章,该方法利用单分子转换定量评估光驱动STM中无任何自由参数的原子级电磁波形11。在该方案中,太赫兹远场聚焦在尖端与样本交界处,从而产生了增强了几个数量级的场。为了以原子尺度分辨率定量评估实际的太赫兹波形,作者将单个分子的酞菁镁(MgPc)引入了隧道间隙。
众所皆知,仅当从局部电场E(t)导出的电压V(t)超过临界阈值时,吸附在铜基板上的氯化钠上的酞菁镁(MgPc)的单个分子才能在两个等效几何形状之间来回切换12。如图1a所示,这种切换机制可作为原子级电压探针。请注意,即使在极弱的穿隧条件下,具有低重复频率的激光脉冲,该单分子的切换统计数据也会发生,并且它适合作为灵敏测量来绘制光驱动STM中的局部场。
为了确保超快的时间分辨率,使用了由麦克森干涉仪生成的两个相同的具有延迟时间τ的THz波形对:弱测试波形VNF(t)和延迟的强闸门波形Vgate(t –τ)。藉由干扰相对于延迟时间在近场中的这两个波形,总电压Vpeak(τ)的峰值可以跟追踪瞬时波形VNF。当尖端限制的峰值电压Vpeak达到〜1.2 V,其中峰值场进入酞菁镁(MgPc)的最低未占分子轨域(LUMO)时,电子穿隧在统计学上会激发翻转事件,然后记录每个激光发射的切换速率p与峰值电压Vpeak的关系。由于LUMO状态的穿隧共振的线宽变宽,因此峰值电压的不同值会引起不同的穿隧速率。如图1b所示,切换速率p随着峰值电压的增加而立即增加,然后达到饱和,其开始行为很容易给出相对于尖端限制的峰值电压Vpeak的p校准曲线;也就是说,导数dp / dVpeak表示透过稳态STM测量的差分电导dI / dV的模拟。因此,透过将dp / dVpeak数据(灰色,图1b)与通过稳态STM(蓝色,图1b)获得的共振曲线进行比较,尖端限制的峰值电压Vpeak可以以伏特为单位直接获得,没有任何自由参数。随后,透过利用反向的起始曲线Vpeak(p),可以在通道接点两端实现局部峰值电压的恢复。
透过根据VNF(t)和Vgate(t –τ)之间的延迟时间τ测量切换速率p,可以提取出迭加波形的峰值电压Vpeak(τ),并且可以得出目标波形VNF(t)是用飞秒时间和原子空间分辨率获得的。检测到的电压波形如图1c所示。检测到的波形类似于通过电光采样对入射远场波形进行的测量。然而,观察到两个波形之间的相位和总线形上的差异,这表明地确定近场波形的重要性。注意,原位方法可以在没有任何自由参数的情况下找回局部场波形,因此适用于各种系统,而与尖端采样条件无关。
为了全面了解所获得的局部场波形,基于经典电动力学和依时密度函数理论(time-dependent density functional theory, TDDFT)的基础上进行了两次模拟。在经典电动力学模拟的情况下,透过用有限元素计算求解马克士威方程组来计算场传播,然后获得尖端限制电压波形。结果,透过比较测得的波形(数据点,图1c)和计算的波形(实线,图1c)可以看出,透过模拟可以很好地再现观察到的波形的总体特征。另一方面,用或不用酞菁镁(MgPc)分子建模的TDDFT计算揭示了超快穿隧过程的量子力学描述的重要性,该过程在时空上改变了局部场。然而,在每个脉冲只有不到一个电子穿隧电位障壁的情况下,穿隧过程不会干扰电压瞬变,从而导致测量波形和经典计算波形之间的一致性,如图1c所示。但是,当每个脉冲有多个电子穿隧电位障壁时,作者预测应考虑穿隧过程的量子力学动力学。
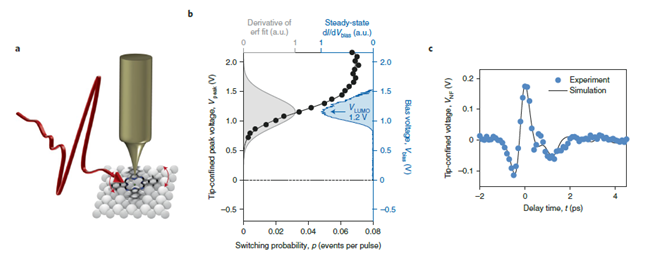
|
图1、近场波形采样。(a)引入光波驱动的STM中的单分子转换的示意图。(b)由尖端限制的峰值电压引起的转换机率p。峰值电压的不同值导致不同的穿隧速率,并且其起始行为给出了作为Vpeak函数的p的校准。(c)检测到的尖端限制电压波形的实验数据,与基于经典电动力学的仿真显示出很好的一致性。面板b,c经参考文献11(施普林格•自然公司Springer Nature Ltd.)许可改编。 |
总之,Dominik Peller及其同事的工作展示了使用单分子转换执行原子尺度的电磁波形采样的能力。由于切换事件统计地发生在峰值电压达到分子共振时,并且无需任何自由参数即可轻松获取局部波形,因此该方法成为光波驱动STM中原位测量的强大工具。基于TDDFT计算的量子力学穿隧过程的全面研究,对于理解原子尺度的光-物质相互作用也有很大的帮助13。因此,这里提出的概念不仅在场驱动显微镜中具有根本的重要性,而且对于探索超快原子尺度电子学也至关重要。
参考资料: |
Jun Takeda & Ikufumi Katayama, “Waveform sampling on an atomic scale,”
Nature Photonics volume
15, 70-71(2021)
https://doi.org/10.1038/s41566-020-00753-z
DOI: s41566-020-00753-z
|
参考文献: |
1. Corkum, P. B. & Krausz, F.
Nat. Phys. 3, 381–387 (2007).
2. Dombi, P. et al. Rev. Mod.
Phys. 92, 025003 (2020).
3. Garg, M. & Kern, K.
Science 367, 411–415 (2020).
4. Cocker, T. L. et al.
Nat. Photon. 7, 620–625 (2013).
5. Jelic, V. et al. Nat. Phys. 13, 591–598 (2017).
6. Cocker, T. L., Peller, D., Yu, P., Repp, J. & Huber, R.
Nature 539,
263–267 (2016).
7. Yoshioka, K. et al. Nat. Photon. 10, 762–765 (2016).
8. Wang, K., Mittleman, D. M., van der Valk, N. C. J. & Planken, P.
C. M. Appl. Phys. Lett. 85, 2715–2717 (2004).
9. Yoshioka, K. et al. Nano Lett.
18, 5198–5204 (2018).
10. Yoshida, S. et al. ACS Photon.
6, 1356–1364 (2019).
11. Peller, D. et al. Nat. Photon. https://doi.org/10.1038/s41566-020-
00720-8 (2020).
12. Peller, D. et al. Nature
585, 58–62 (2020).
13.
Jestädt, R., Ruggenthaler, M.,
Oliveira, M. J. T., Rubio, A. &
Appel, H. Adv. Phys.
68, 225–333 (2019).
|
|
|
|
|
 |
|
|
|
|
|
|
|
|
|
 |
|
|
|
 |
|
 |
|